Impacts
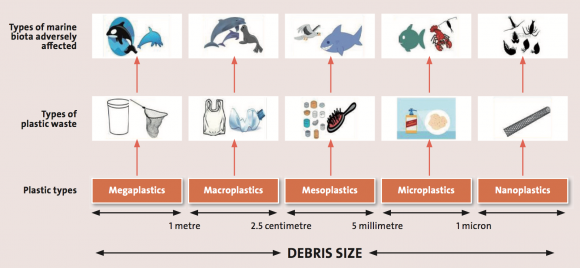
Diagram from Chatterjee and Sharma (2019) showing how different size classes of plastic impact different sizes of marine life.
About
Since the first reports on the prevalence of small plastics fragments in the environment in 1970s (Carpenter & Smith 1972), numerous studies around the World have shown that plastics are pervasive in nearly every marine habitat (Auta et al. 2017; Law 2017). The relatively small size of most plastic debris (GESAMP 2015) facilitates their ingestion by a wide range of organisms, from zooplankton and bivalves to fish, seabirds, marine mammals, and even humans (Andrady et al. 2011; Cole et al. 2015; Lusher et al. 2017). Plastic consumption can have major adverse effects on the nutrition, biology, and physiology of organisms through damage or blocking of the digestive tract, false satiation, reduction in growth and reproduction, and potential toxicity from absorbed pollutants (Moore 2008; Cole et al. 2011; Sutton et al. 2016; Fossi et al. 2016). These impacts can, in turn, have cascading impacts on the structure and function of entire ecosystems through changes in the distribution and abundance of affected organisms and alterations of organismal interactions (Galloway et al. 2017).
Filter-feeding bivalves, such as oysters, have become a primary research focus for studying the impacts of plastic pollution on marine ecosystems given their abundance in heavily human-impacted coastal ecosystems and their ability to process large volumes of water during feeding (∼5-25 L seawater h-1; Korringa 1952), and their potential to serve as direct vectors of microplastics to humans through consumption (Van Cauwenberghe & Janssen 2014; Rochman et al. 2015). In experimental studies, ingestion of microplastics has strong negative effects on bivalve reproduction, physiology, and health through false satiation and mechanical damage to digestive systems (e.g. Sussarellu et al. 2016; Van Cauwenberghe & Janssen 2014). Filter feeders form the base of marine food webs and can connect microplastic pollution to higher trophic levels, both directly, through trophic interactions, and indirectly, through disruption to their growth, survival, and reproduction. However, there is an emerging divergence in opinion of the actual severity of impacts that bivalves face in the natural environment (e.g. Egbeocha et al. 2019; Lenz et al. 2016; Shumway et al. 2018).
Studies in the natural environment, or exposure experiments that use naturally occurring levels of microplastic remain a rarity, largely due to logistical challenges and lack of supporting field measurements. Many dose-response experiments have been conducted with microplastic concentrations that are several orders of magnitude higher than those reported within field studies. Contradictory studies are emerging that demonstrate sublethal, but potentially additive, responses when organisms are exposed to environmentally relevant concentrations of microplastics (e.g. Raineri et al. 2018; Revel et al. 2019; Weber et al. 2018). Such responses are perhaps not surprising given that many bivalve species have mechanisms to cope with the presence of non-food particles in the water column (particularly sediments), including rejection as pseudofeces prior to ingestion, preferential selection of particles, post-ingestive selection in the stomach, and differential absorption in the gut (Ward & Shumway, 2004).
Microplastics likely represent a significant stress-factor for bivalve species, due to both mechanical impacts from ingestion and impacts on their physiology (e.g. Kazmiruk et al. 2018; Smith et al. 2018). These direct impacts can alter growth, energy acquisition, and immune response, which may in turn lead to increased susceptibility to other stressors such as pollutants, disease, predation and climate change (Nelms et al. 2019). Microbial biofilms that rapidly colonize plastics in the marine environment may further exacerbate these impacts by facilitating the transfer of pollutants and pathogens from the environment to consumers (Kirstein et al. 2016). The rapid development of biofilms on plastics suggests that plastics may also play a critical, yet understudied, role in disease spread dynamics (Lamb et al., 2018; Curren & Leong 2019). The reduced fitness of organisms consuming microplastics may lead to lowered immunoresistance and higher potential for infection (e.g. Browne et al. 2008). Understanding the role that microplastics play in disease transmission and pathogenesis is critical to monitoring the health and function of oysters and the humans that consume them. Protozoan and bacterial pathogens (e.g. Perkinsus marinus Dermo, Juvenile Oyster Disease) have a long history of heavily impacting oyster populations and the ecosystem goods and services that oysters provide (Lafferty et al., 2015). Oysters, which are often consumed raw, can also carry the human pathogens, such as Vibrio parahaemolyticus and V. vulnificus, which cause an estimated 80,000 illnesses and 100 deaths in the US each year (https://www.cdc.gov/vibrio/faq.html).
Citations
Andrady, A.L. (2011). Microplastics in the marine environment. Marine Pollution Bulletin 62, 1596–1605.
Auta, H.S., Emenike, C.U., and Fauziah, S.H. (2017). Distribution and importance of microplastics in the marine environment: A review of the sources, fate, effects, and potential solutions. Environment International 102, 165–176.
Browne, M.A., Dissanayake, A., Galloway, T.S., Lowe, D.M., and Thompson, R.C. (2008). Ingested Microscopic Plastic Translocate to the Circulatory System of the Mussel, Mytilus edulis (L.). Environmental Science & Technology 42, 5026–5031.
Carpenter E.J. and Smith K.L. Jr. (1972). Plastics on the Sargasso sea surface. Science 175, 1240-1241
Chatterjee S. and Sharma S. (2019) Microplastics in our oceans and marine health, Field Actions Science Reports [Online], Special Issue 19 | Online since March 2019, connection on 21 April 2020. URL : http://journals.openedition.org/factsreports/5257
Cole, M., Lindeque, P., Halsband, C., and Galloway, T.S. (2011). Microplastics as contaminants in the marine environment: A review. Marine Pollution Bulletin 62, 2588–2597.
Cole, M., Webb, H., Lindeque, P.K., Fileman, E.S., Halsband, C., and Galloway, T.S. (2015). Isolation of microplastics in biota-rich seawater samples and marine organisms. Scientific Reports 4. 4528.
Curren, E., and Leong, S.C.Y. (2019). Profiles of bacterial assemblages from microplastics of tropical coastal environments. Science of The Total Environment 655, 313–320.
Egbeocha, C.O., Malek, S., Emenike, C.U., Milow, P. (2018). Feasting on microplastics: ingestion by and effects on marine organisms. Aquatic Biology 27, 93-106
Fossi, M.C., Marsili, L., Baini, M., Giannetti, M., Coppola, D., Guerranti, C., Caliani, I., Minutoli, R., Lauriano, G., Finoia, M.G., et al. (2016). Fin whales and microplastics: The Mediterranean Sea and the Sea of Cortez scenarios. Environmental Pollution 209, 68–78.
Galloway, T.S., Cole, M., and Lewis, C. (2017). Interactions of microplastic debris throughout the marine ecosystem. Nature Ecology &Amp; Evolution 1, 0116.
GESAMP (Joint Group of Experts on the Scientific Aspects of Marine Environmental Protection), (2015). Sources, fate and effects of Microplastics in the Marine Environment: A Global Assessment.
Kazmiruk, T.N., Kazmiruk, V.D., and Bendell, L.I. (2018). Abundance and distribution of microplastics within surface sediments of a key shellfish growing region of Canada. PLOS ONE 13, e0196005.
Kirstein, I.V., Kirmizi, S., Wichels, A., Garin-Fernandez, A., Erler, R., Löder, M., and Gerdts, G. (2016). Dangerous hitchhikers? Evidence for potentially pathogenic Vibrio spp. on microplastic particles. Marine Environmental Research 120, 1–8.
Lamb, J.B., Willis, B.L., Fiorenza, E.A., Couch, C.S., Howard, R., Rader, D.N., True, J.D., Kelly, L.A., Ahmad, A., Jompa, J., et al. (2018). Plastic waste associated with disease on coral reefs. Science 359, 460–462.
Lafferty, K.D., Harvell, C.D., Conrad, J.M., Friedman, C.S., Kent, M.L., Kuris, A.M., Powell, E.N., Rondeau, D., and Saksida, S.M. (2015). Infectious Diseases Affect Marine Fisheries and Aquaculture Economics. Annual Review of Marine Science 7, 471–496.
Law, K.L. (2017). Plastics in the Marine Environment. Annual Review of Marine Science 9, 205–229.
Lenz, R., Enders, K., and Nielsen, T.G. (2016). Microplastic exposure studies should be environmentally realistic. Proceedings of the National Academy of Sciences 113, E4121–E4122.
Lusher, A.L., Welden, N.A., Sobral, P., and Cole, M. (2017). Sampling, isolating and identifying microplastics ingested by fish and invertebrates. Analytical Methods 9, 1346–1360.
Moore, C.J. (2008). Synthetic polymers in the marine environment: A rapidly increasing, long-term threat. Environmental Research 108, 131–139.
Nelms, S.E., Barnett, J., Brownlow, A., Davison, N.J., Deaville, R., Galloway, T.S., Lindeque, P.K., Santillo, D., and Godley, B.J. (2019). Microplastics in marine mammals stranded around the British coast: ubiquitous but transitory? Scientific Reports 9. 1075
Rainieri, S., Conlledo, N., Larsen, B.K., Granby, K., and Barranco, A. (2018). Combined effects of microplastics and chemical contaminants on the organ toxicity of zebrafish (Danio rerio). Environmental Research 162, 135–143.
Revel, M., Lagarde, F., Perrein-Ettajani, H., Bruneau, M., Akcha, F., Sussarellu, R., Rouxel, J., Costil, K., Decottignies, P., Cognie, B., et al. (2019). Tissue-Specific Biomarker Responses in the Blue Mussel Mytilus spp. Exposed to a Mixture of Microplastics at Environmentally Relevant Concentrations. Frontiers in Environmental Science 7, 33.
Rochman, C.M., Tahir, A., Williams, S.L., Baxa, D.V., Lam, R., Miller, J.T., Teh, F.-C., Werorilangi, S., and Teh, S.J. (2015). Anthropogenic debris in seafood: Plastic debris and fibers from textiles in fish and bivalves sold for human consumption. Scientific Reports 5. 14340
Shumway, S.E., Ward, J.E., and Mladinich, K. (2018). The Microplastics and Shellfish Media Frenzy: Stop The Train, We Want To Get Off! East Coast Shellfish Growers Association Newsletter 16–17.
Smith, M., Love, D.C., Rochman, C.M., and Neff, R.A. (2018). Microplastics in Seafood and the Implications for Human Health. Current Environmental Health Reports 5, 375–386.
Sutton, R., Mason, S.A., Stanek, S.K., Willis-Norton, E., Wren, I.F., and Box, C. (2016). Microplastic contamination in the San Francisco Bay, California, USA. Marine Pollution Bulletin 109, 230–235.
Sussarellu, R., Suquet, M., Thomas, Y., Lambert, C., Fabioux, C., Pernet, M.E.J., Le Goïc, N., Quillien, V., Mingant, C., Epelboin, Y., et al. (2016). Oyster reproduction is affected by exposure to polystyrene microplastics. Proceedings of the National Academy of Sciences 113, 2430–2435.
Van Cauwenberghe, L., and Janssen, C.R. (2014). Microplastics in bivalves cultured for human consumption. Environmental Pollution 193, 65–70.
Ward, E. J., and Shumway, S.E. (2004). Separating the grain from the chaff: particle selection in suspension- and deposit-feeding bivalves. Journal of Experimental Marine Biology and Ecology 300, 83–130.
Weber, A., Scherer, C., Brennholt, N., Reifferscheid, G., and Wagner, M. (2018). PET microplastics do not negatively affect the survival, development, metabolism and feeding activity of the freshwater invertebrate Gammarus pulex. Environmental Pollution 234, 181–189.